Let $f: X\longrightarrow Y$ be a function. The continuity of $f$ is then determined by the topologies on $X$ and $Y$. To be precise, $f: X\longrightarrow Y$ is continuous on $X$ if for every open set $U\subset Y$ $f^{-1}(U)$ is open in $X$. But if you have a clear idea of what class of functions, say $\{f_\alpha:X\longrightarrow Y|\alpha\in\mathscr{A}\}$, should be continuous, you can also define a topology on $X$ that makes $f_\alpha :X\longrightarrow Y$ continuous for all $\alpha\in\mathscr{A}$ as Sam Walters mentioned on his tweet. Here is how. Let $$\mathscr{S}=\{f_{\alpha}^{-1}(U)| \mbox{$U$ is open in $Y$}, \alpha\in\mathscr{A}\}$$
Since $\bigcup\{G|G\in\mathscr{S}\}=X$, $\mathscr{S}$ is a subbase for a topology in $X$. (Note: $\mathscr{S}$ is not a base for a topology in $X$ unless $\mathscr{A}$ is a singleton set.) Denote by $\tau(\mathscr{S})$ the topology generated by the subbase $\mathscr{S}$. Then $\tau(\mathscr{S})$ is the smallest topology on $X$ that makes $f_\alpha :X\longrightarrow Y$ continuous for all $\alpha\in\mathscr{A}$. The reason is any topology on $X$ that makes $f_\alpha :X\longrightarrow Y$ continuous for all $\alpha\in\mathscr{A}$ would include $\mathscr{S}$. We have in fact seen a similar idea which is Tychonoff product topology. Consider the cartesian product
$$\prod_{\alpha\in\mathscr{A}}X_\alpha=\{c:\mathscr{A}\longrightarrow\bigcup_{\alpha\in\mathscr{A}}X_\alpha\}$$
of topological spaces $(X_\alpha,\tau_\alpha)$, $\alpha\in\mathscr{A}$. The topology we want is the one that makes the projection maps $\pi_\alpha :\prod_{\alpha\in\mathscr{A}}X_\alpha\longrightarrow X_\alpha$ for all $\alpha\in\mathscr{A}$ continuous in particular the smallest one. Let $$\mathscr{S}=\{\pi_\alpha^{-1}(U_\alpha)|U_\alpha\in\tau_\alpha,\forall\alpha\in\mathscr{A}\}$$
Then $\mathscr{S}$ is a subbase for a topology called the Tychonoff product topology. This is the smallest topology that makes the projection maps continuous. The projection maps are also open.
Given a surjective function $p: X\longrightarrow Y$ from a topological space $X$ onto a set $Y$, we can also define a topology on $Y$ that makes $p$ continuous: $U\subset Y$ is open if and only if $p^{-1}(U)$ is open in $X$. This topology on $Y$ automatically makes $p$ continuous. Moreover it is the largest topology on $Y$ that makes $p$ continuous. This topology is called the identification topology. The identification topology can be used to get a new topological space (identification space) from an old one. More specifically, it can be defined on a partition of $X$ or equivalently a quotient set of $X$ modulo an equivalence relation and it makes the canonical projection $\pi$ continuous. For example, let $X$ be the unit square $[0,1]\times[0,1]$ in $\mathbb{E}^2$ with the subspace topology. Partition $X$ into the following subsets:
- $\{(0,0),(1,0),(0,1),(1,1)\}$ i.e. the set of four corner points.
- $\{(x,0),(x,1)|0<x<1\}$
- $\{(0,y),(1,y)|0<y<1\}$
- $\{(x,y)\}$ where $0<x<1$, $0<y<1$.
The resulting identification space is the torus which is homeomorphic to $S^1\times S^1$ as shown in Figure 1.
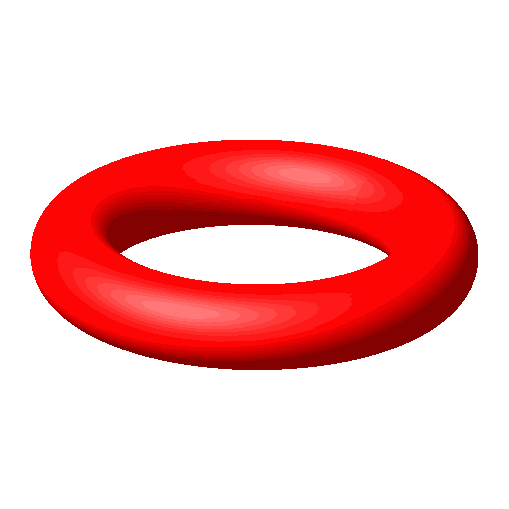
Figure 1. Clifford Torus S^1 x S^1
References:
- M. A. Armstrong, Basic Topology, Springer-Verlag, 1983
- Benjamin T. Sims, Fundamentals of Topology, Collier Macmillan